Extract of the fruits of Vitex payos on lipid peroxidation and oxidative stress in diabetic rats
- Nicol Jeyacheya
- Dec 5, 2023
- 29 min read
By
Solomon T. Muradzikwa
ABSTRACT
Diabetes Mellitus describes a metabolic disorder with different etiologies which is characterized by persistent hyperglycemia and disturbances of carbohydrate, protein and fat metabolism resulting from defects in insulin secretion, insulin action or both. This is associated with damage and failure of various organs such as the liver, the kidney, the blood vessels, and the heart. Although traditional medicines have been used in food and herbs to control this condition, their specific physiological action has not been explored for all plant species. The aim of this study was to determine the hypoglycemic activity, and effects of the aqueous extract of fruits of Vitex payos on lipid peroxidation and oxidative stress in alloxan-induced diabetic rats. Male Sprague-Dawley rats weighing 193.23 ± 62.02g were induced with diabetes using alloxan monohydrate intraperitoneally and treated with different concentrations of extract of fruits of Vitex payos, distilled water and glibenclamide over a 30 day period. Blood glucose was measured, the livers were harvested for analysis and blood serum was also collected for analysis using specific protocols. The fruit extract did not have any effect on glucose levels of normal rats but had significant effect of lowering the glucose levels of diabetic rats with the 800mg/kg concentration the most effective (6.8 ± 0.79mmol/dL). Activity of the antioxidant enzymes SOD and peroxidase significantly increased upon treatment with extracts, with the 800mg/kg concentration showing the greatest effect. The diabetic rats on distilled water treatment showed the highest levels of MDA and catalase activity. The serum levels of AST and ALT were lowest in the control rats and the diabetic rats treated with glibenclamide. Aqueous Vitex payos fruit extract increases the activity of antioxidant enzymes and lowers blood glucose levels. This research significantly increases the need to identify more local plant species that can alleviate the effects of diabetes.
LIST OF ABBREVIATIONS
ALP Alanine Phosphatase
ALT Alanine aminotransferase
ANOVA Analysis of variance
AST Aspartate aminotransferase
BSA Bovine serum albumin
CAT Catalase
CB Coomassie brilliant blue
DM Diabetes mellitus
DMSO Dimethyl sulfoxide
DNA Deoxyribonucleic acid
GGTP Gamma-glutamyl transpeptidase
GPXs Glutathione peroxidases
GSH Reduced glutathione
HbAlc Hemoglobin A1C
HDL-c High-density lipoprotein-cholesterol
HIV Human Immunodeficiency virus
IFCC International federation of clinical chemistry
MDA Malondialdehyde
NF-κB Nuclear Factor kappa B
NO Nitrogen oxide
OHA Oral hypoglycemic agents
PUFAs Polyunsaturated fatty acids
RNS Reactive nitrogen species
ROS Reactive oxygen species
SD Standard deviation
SOD Superoxide dismutase
T2DM Type 2 Diabetes Mellitus
TBARS Thiobarbituric acid reactive substances
US United States
WHO World health organization
XOD Xanthine oxidase dismutase
Motivation
Diabetes Mellitus (DM), a most challenging health problem in the 21st century, is a persistent metabolic ailment that is of major public health concern the world over (Seid et al., 2021). Diabetes mellitus is a metabolic condition caused by defects in insulin secretion, action, or both. It is characterized by hyperglycemia and problems in the metabolism of fat, carbohydrates, and lipids. This leads to macro and microvascular complications (Wild et al., 2004). It affects multiple organ systems and combined complications of vascular nature are considered as the major cause of death around the world. Due to dyslipidemia and oxidative stress, patients with diabetes are at a high risk of accelerated development of atherosclerosis. (Engelen et al., 2005).
Artherosclerosis is a disease of the arteries characterised by the deposition of fatty material on their inner walls. Dyslipidemia is defined as having blood lipid levels that are too high or too low. An imbalance between the creation and buildup of reactive oxygen species (ROS) in cells and tissues and a biological system's capacity to detoxify these reactive products results in the phenomena known as oxidative stress.
There are at least four different types of DM. These are type I, type 2, gestational and prediabetes. Of the DM cases reported, almost 90% are attributed to Type 2 DM (T2DM). The prevalence of diabetes ranges between 2-7% of the population, which increases with age to between 10-14% of patients above 40 years. The prevalence of diabetes has risen exponentially in the last decade. Globally, the prevalence for all age groups was predicted to increase from 2.8% in 2000 to 4.4% in 2030.
T2DM is caused by either insulin resistance or β-cell dysfunction, along with intricate interactions between a person's genetic make-up and their environment. The closely regulated generation and secretion of insulin in the pancreas is carried out by cells known as β-cells.
To identify those who are more likely to develop T2DM, several clinical risk factors are typically used. These include fasting blood sugar, triglycerides, high-density lipoprotein cholesterol (HDL-c), and glycosylated hemoglobin (HbA1c), as well as the enzymes aspartate aminotransferase (AST) and alanine aminotransferase (ALT) (Meigs, 2009). The two enzymes AST and ALT have numerous physiological functions and have long been recognized as indicators to determine liver damage (Sookoian and Pirola, 2015). The use of the AST/ALT ratio to predict the chance of future development of T2DM still remains uncertain.
Study background
Several microvascular complicatons arise as a result of T2DM. These include nephropathy (the detereoration of kidney function), retinopathy (disease of the retina of the eye) and neuropathy (disease of one or more peripheral nerves). Macrovascular complications include artherosclerosis and peripheral vascular condition (gangrene and ulceration). Oxidative stress is brought on by persistent hyperglycemia in diabetes, which increases the formation of oxygen free radicals through autoxidation of glucose and glycosylation of proteins. Autoxidation is the term used to describe oxidation caused by interactions with oxygen at room temperature without the need of flame or an electric spark (Foote, 1996).
Particularly membrane lipids, connective tissues, and cell nucleic acids are at considerable risk from free radicals produced by a partial reduction of oxygen in tissues and essential organs. Consequently, enzymes that defend against these reactive oxygen species and repair any DNA damage have developed (Rehman et al., 1992).
Oxidative stress can cause disruptions in normal mechanisms of signalling between or amongst different cells. Chemically, oxidative stress is linked to a rise in the generation of oxidizing species or a sharp drop in the efficiency of antioxidation defenses, including antioxidant enzymes. These enzymes include ALT, AST, glutathione peroxidases (GPXs), catalases, superoxide dismutases (SODs), and four ascorbate-glutathione pathway enzymes (Matamoros et al., 2003).
Hyperglycemia can increase oxidation stress through several pathways. A key mechanism appears to be the hyperglycemic-induced intracellular reactive oxygen species (ROS), which are formed by the proton electromechanical gradient created by the mitochondrial electron transport chain and leading to an increase in superoxide generation (Santos et al., 2018). To further clarify how hyperglycemia increases ROS generation, two additional pathways have been put forth. One includes free glucose being oxidized by a transition metal. The glucose itself triggers an oxidative process that produces free radicals and oxygen ions as well as hydrogen peroxide. The second process entails the transition metal-catalyzed autoxidation of protein-bound Amadori compounds, which results in the production of superoxide and hydroxyl radicals as well as very reactive dicarbonyl molecules.
Research aim
The aim of this project is to determine the effects of the aqueous extract of fruits of Vitex payos on lipid peroxidation and oxidative stress in diabetic rats.
Research objectives
a) To prepare an aqueous extract of the fruit of Vitex payos
b) To prepare a fattening rat feed to be administered over 30 days
c) To induce sugar diabetes in selected male Sprague Dawley rats
d) To treat the experimental animals with prepared aqueous extract, both diabetic and the controls and glibenclamide (a known diabetes mellitus drug)
e) To determine the blood sugar, activity of antioxidant enzymes, and TBARS in blood samples.
Research significance
This research will provide additional insights into the medicinal benefits of mutsubvu (Vitex payos) specifically on its hypoglycemic ability and effects on oxidative stress and lipid peroxidation.
Through this research, the local community will further realize promoting the use of medicial plants, especially the chocolate berry, as a treatment for metabolic injury caused by the condition of Diabetes Mellitus. People and medical research institutions may also consider the chocolate berry as an additional supplement against hyperglycemia as it may have less side effects to conventional drugs, and may offer some alternatives to poor income societies.
Moreover, the analysis that is presented in this study will convey valuable information for future research that will explore the various medicinal benefits of the chocolate berry, its related family species and other medicinal plants that have been found locally in our societies.
Vitex payos fruit is fleshy, shiny black, ovoid, and cupped in a persistent calyx. It has been assessed for nutrient content. It contains useful quantities of potassium, manganese, phosphorous, vitamin B3, vitamin B6, and vitamin C. Sodium, magnesium, and calcium are present in minute quantities. The fruits are available between April and July during the dry season.
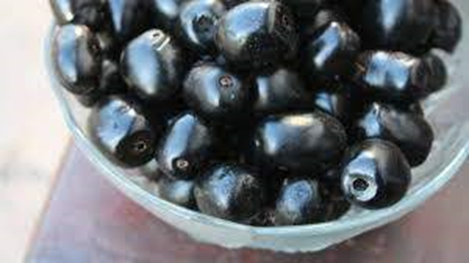
Figure 2.4. Fruit of Vitex payos.
Methodology
Preparation of materials
Collection and identification of the fruit
The ripe fruits of Vitex payos were collected from Nehumba Village in Rusape,Manicaland, Zimbabwe. They were then washed with distilled water and stored in a freezer at -20oC until use. The sample of fruits was identified and attested by a botanist at the National Botanical Gardens of Zimbabwe.
Preparation of rat feed
The rats were pre-disposed to DM by a high fat and sugar content feed prepared from a formulation adapted from Taderera et al. (2014). The feed was prepared by mixing ground standard rat feed (Profeeds, Zimbabwe, 48%) as the carbohydrate, soya bean oil (8%) as the unhealthy oil, and condensed milk (44%) for the provision of high sugar. The components were mixed, and a little distilled water was added to combine the ingredients (100-150ml). The mixture was moulded by hand into long cylindrical shapes and cut using a small knife into manageable sizes for the rats to consume. It was then baked at 100oC for 48 hours until completely dry.
Preparation of the rats
Fifty normal healthy male Sprague-Dawley rats aged 4 weeks were housed under standard environmental conditions in clean cages at the Animal House of the University of Zimbabwe. Light and dark were alternated at 12-hour periods with temperatures maintained at 25oC. The initial average weight of the rats was 193.23 ± 62.02g. The animals were randomly selected and individually marked for identification and kept for 5 days prior to individual measurements being taken to acclimatize to the laboratory conditions. A total of 10 groups of 5 animals each were used for the experiment. Initially, 6 groups were fed the high-fat and sugar-formulated diet, and 1 group was fed with normal rat pellets (Profeeds, Zimbabwe) for 30 days. Both water and food were provided ad libitum for all 7 groups.
Table: Grouping of the rats
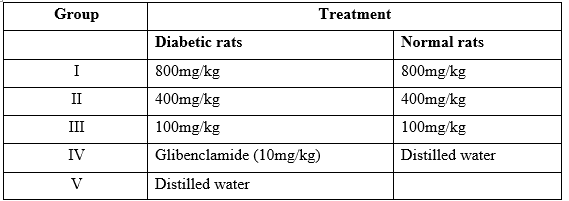
Alloxan-induced diabetic rats were grouped into 5 groups for the 5 different treatments. The control rats were also grouped into 4 groups for the 4 different treatments as shown above.
Preparation of samples
The frozen fruits of V. payos were allowed to thaw at room temperature overnight. The peels were removed by hand and the soft dark fleshy pulp was removed from the stone using a small knife and stored in a refrigerator until the required amount was obtained in a 1L beaker. The pulp was then heated in an oven at 40oC until a constant weight was attained. The dried material was ground to a powder and stored at room temperature in a brown paper bag for further use.
Extraction of Phytochemicals
Three separate 40g samples of the powdered pulp of V. payos were hydrated with 200ml of distilled water and shaken for 48 hours at 150rpm (Thermolyne Rotomix shaker M50820, Iowa, USA) at room temperature. This was to extract the water-soluble compounds. The mixtures were then centrifuged at 3000rpm for 20 minutes and the supernatants were combined to make 320ml called Extract 1. This was sent for freeze-drying.
The remaining pellets were redissolved in 200ml of distilled water and shaken for 48 hours for Extract 2. This was centrifuged at 3000rpm for 20 minutes and the supernatants were combined and stored in a refrigerator for future use.
The concentrations of the remaining supernatant of Extract 1 and the supernatant of Extract 2 were calculated.
Preparation of alloxan-monohydrate and inducing diabetes
To induce diabetes in the experimental animals, alloxan monohydrate stock solution (110mg/kg) was freshly prepared by dissolving 3.3g in 30ml of distilled water. A total of 30 rats were induced with a single intraperitoneal injection. After 4 days, blood was drawn using a tail vein puncture, and glucose concentrations were determined using a Direct and Quick Care® glucometer. Glucose concentrations of 11mmol/dL and above were considered as indicating a diabetic state (Taderera et al, 2014).
Preparation of glibenclamide
60mg of glibenclamide tablets were crushed in a mortar and pestle and dissolved in 20ml of dimethyl sulfoxide (DMSO) to make a stock solution of 10mg/kg.
Administration of treatments
To mimic the intended route of administration in humans, the different concentrations of aqueous fruit extract of V. payos (800mg/kg, 400mg/kg, 100mg/kg), glibenclamide, and distilled water were administered once daily at the same time (10 am) using the oral gavage method. Weight was measured once a week using the weight balance available at the Animal House and glucose readings were taken using a Direct and Quick Care® glucometer once per week and recorded. This was done over 30 days.
Collection of livers and blood for serum sample
The rats were stunned in preference to using available anesthesia. This is because anesthesia interferes with MDA present in the liver which is to be analyzed for lipid peroxidation. Blood was collected from all the animals using a 23G1 needle and 5ml syringe via the cardiac puncture method. The blood was immediately placed in 5ml vacuum tubes coated with a clot activator and left to stand for 5 minutes. The tubes were then centrifuged for 2 minutes at 2500rpm, and the serum was individually collected as supernatant and immediately placed in a freezer at -20oC. The animals were then cervically dislocated and immediately dissected to collect the livers. The cervical dislocation technique aims to provide the animal with a fast and painless death.
A 1% saline solution was injected into the heart to wash red blood cells from the liver before cutting it out. Each liver was weighed and then stored in a freezer at -20oC for further analysis.
Enzyme assays
The following antioxidant enzymes were assayed from the liver samples: catalase (CAT), superoxide dismutase (SOD), and peroxidase. Alanine aminotransferase and aspartate aminotransferase were assayed from the blood serum. The concentration of malondialdehyde was also determined from the samples. Each liver was thawed at room temperature for a few minutes and then homogenized in 10ml of cold 100mM phosphate buffer pH 6.8. The homogenate was then ultra-centrifuged at 10000rpm for 20 minutes at 4oC. The supernatant was stored in the freezer at -20oC and the pellet was also stored under the same conditions.
Preparation of phosphate buffer pH 6.8
To 800ml distilled water was added 13.124g of sodium phosphate dibasic heptahydrate. 7.043g of sodium phosphate monobasic monohydrate was then added to the same solution and mixed. The final desired pH was adjusted using HCl or NaOH and distilled water was added to make the final volume 1L. The buffer was then refrigerated to keep it cold.
Catalase activity
The rate of enzyme activity was obtained by measuring the change in absorbance (using a UV-Vis spectrophotometer BK-D560) during 3 minutes at 240nm in 3ml of reaction mixture consisting of 100mM phosphate buffer pH 6.8 (2ml), 30mM hydrogen peroxide (0.5ml) and 0.5ml diluted extract. The enzyme extract was diluted 2-fold. Phosphate buffer (100mM, 2.5ml) pH 6.8 and 0.5ml of diluted enzyme extract were used as the control. Catalase activity was expressed in units per milligram of protein per milliliter.
1 catalase unit = amount of enzyme to decompose 1 millimole H2O2 per minute at 25oC and pH 7.
SOD activity
Tris-EDTA buffer (0.0012mM, pH 8.5, 2.8ml) and pyrogallol (2mM, 150µl) were mixed in a cuvette and scanned for 3 minutes at 420nm in a UV-Vis spectrophotometer as the control. We allowed at least 30 seconds for the reaction mixture to reach a stable reaction rate.
The test sample consisted of Tris-EDTA buffer (0.0012mM, pH 8.5, 2.8ml), pyrogallol (2mM, 150µl), and 50µl diluted enzyme extract mixed in a cuvette. They were scanned for 3 minutes to measure the percentage inhibition of pyrogallol autoxidation after an initial 30-second allowance for the reaction mixture to reach a stable reaction rate.
One unit of SOD activity is the amount of enzyme that inhibits the rate of autoxidation of pyrogallol by 50% and is expressed as units/mg protein/ml.
Pyrogallol was always kept on ice before use and away from sunlight by storing the prepared sample in foil.
Determination of lipid peroxidation
Lipid peroxidation is a complex process in which PUFAs are subjected to attack via oxygen-derived free radicals resulting in the formation of lipid hydroperoxides such as MDA. MDA is broadly used as a marker of oxidative lipid damage because of environmental stress. One molecule of MDA reacts with 2 molecules of TBA forming a pink-coloured complex having an absorption maximum at 532nm in a UV spectrophotometer.
A 1% solution of TBA was prepared by dissolving 1g in 80cm3 in distilled water. The solution was then topped up to 100cm3 with distilled water, then heated at 50oC for 45 minutes. This dissolves the TBA producing a yellowish liquid.
To 2ml of the diluted enzyme extract sample, 0.5ml of the 1.0% thiobarbituric acid was added, followed by heating at 95oC for 1 hour to separate the pink-coloured malondialdehyde. The samples were then immediately placed on ice for 2 minutes to stop any further reaction. They were then centrifuged at 6000rpm for 20 minutes and the supernatant was collected for analysis.
A UV-Vis spectrophotometer was used and a range was set from 532nm to 600nm, taking the exact readings at 532nm and 600nm.
Protein concentration determination
The Bradford assay was used to quantitate the amount of protein in the diluted liver samples collected from the rats, based on a colorimetry method. The dye used was Coomassie Brilliant Blue (CB).
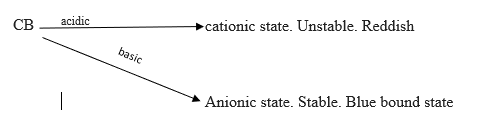
The intensity of the colour shows how much protein is present. Proteins bind to the dye via their amino acids (bound state) and so the intensity of the blue colour indicates the amount of protein present.
Coomassie Brilliant blue (12.5mg) was dissolved in 6.25ml of 95% ethanol. The solution was then mixed with 12.5ml of 85% phosphoric acid and made up to 125ml with distilled water. The reagent was filtered through Whatman filter paper and stored in a flask covered in foil at room temperature.
For the protein standard Bovine Serum Albumin (BSA) 100µl/mg in distilled water was used as the stock solution. For the calibration curve, duplicate volumes of 10, 20, 40, 60, 80, and 100µl of BSA standard solution were placed into cuvettes, and the volumes were adjusted to 100µl using distilled water. For the reagent blank 100µl of distilled water was used. 1ml of protein reagent was added to each tube and mixed gently, but thoroughly. Absorbance values were measured and recorded at 595nm, 3 minutes after the addition of the protein reagent.
A standard curve was plotted using the concentration of protein (mg/ml) against absorbance for the protein standard (BSA). Each diluted sample (100µl) was placed in a cuvette and 1ml of protein reagent was added. The absorbance values were recorded after 3 minutes at 595nm. The standard curve of the BSA was then used to determine concentrations of diluted liver samples that corresponded to their absorbance values.
AST and ALT concentrations
The serum collected for each rat was used to assay for concentrations of aspartate aminotransferase (AST) and alanine aminotransferase (ALT). The samples were kept frozen and taken to Interpath Medical Laboratories (Five Avenue, Harare, Zimbabwe) for analysis. AST was measured using an Aspartate Aminotransferase Kit (IFCC Method) and ALT using an Alanine Aminotransferase Kit (IFCC Method) in a BS200 Biochemistry analyzer.
Results
Feeding of Rats With High Fat and Sugar Diet
Over the 30 days, the mass of rats increased (figure 4.1). The initial average weight of all the rats combined was 193.23 ± 62.02g. The average weight of the rats at 30 days was 242.44 ± 39.74g for those on the high fat and sugar content diet and 134.8 ± 20.98g for those on standard rat feed. The difference in weight was significant between the two groups (unpaired t-test, P=0.000003).
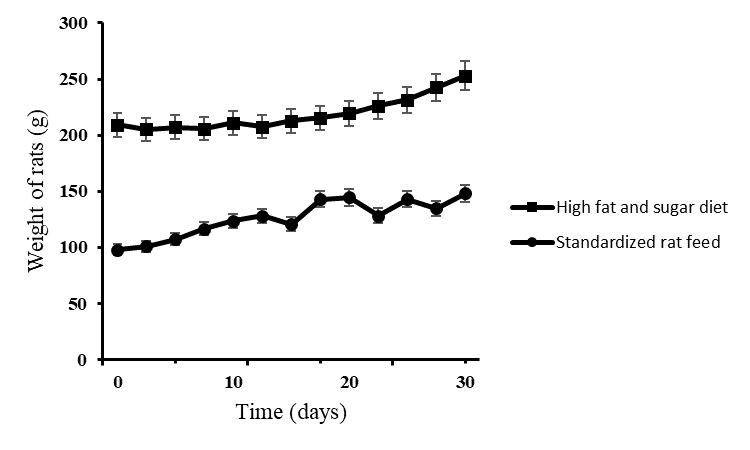
Figure. Average body weights of rats that were fed on high fat and sugar diet and standardized rat feed over the course of 30 days. The results are the average weight for 35 rats ± SD.
Blood Glucose Levels in Diabetic Rats Exposed to Different Treatments
The treatments given to the rats were 5 in total. These were glibenclamide, distilled water and 3 extract concentrations of 100mg/kg, 400mg/kg and 800mg/kg. Rats in the negative control group were given distilled water and some died on day 2 and some on day 4 before the second measurement of blood glucose. The glucose level of the rats treated with distilled water continued to increase from day 1 through to day 30 (figure 2) from an initial of 23.78 ± 18.74 mmol/dL to 35.40 ± 5.39 mmol/dL. Glibenclamide treatment showed the most rapid decrease in blood glucose from 18.43 ± 18.68 mmol/dL to 8.13 ± 2.44 mmol/dL. Of the 3 extract concentrations, the 800mg/kg extract showed the greatest effect of lowering blood glucose to 6.8 ± 0.79 mmol/dL over the 30 days measured. The 100mg/kg extract lowered the blood glucose level to 16.18 ± 15.97 mmol/dL and the 400mg/kg to 16.03 ± 16.25 mmol/dL. Significant differences of the means were accepted at p<0.05. From the results the differences in the means were significant (ANOVA: single factor, P = 0.00000015).
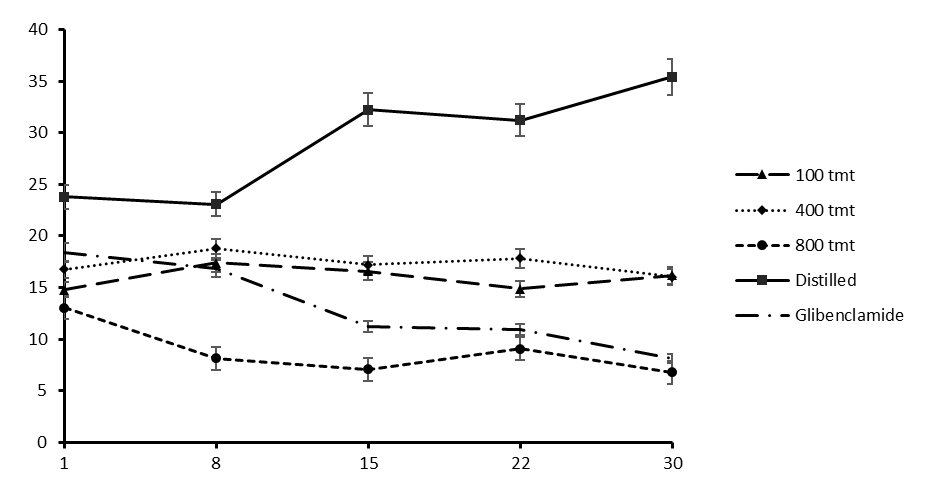
Figure. Levels of blood glucose in the diabetic rats treated with crude aqueous extract of V. payos fruits, distilled water and glibenclamide. Results are given as mean ± SD for the rats in each respective group.
Blood Glucose Levels of Normal Rats Exposed to Different Treatments
There was no significant difference (figure 3) among the normal rats treated with distilled water and the three concentrations (100mg/kg, 400mg/kg and 800mg/kg) of V. payos aqueous fruit extract (p>0.05). The results are given as mean ± SD for the rats in each specific group. All the treatment groups maintained a constant blood glucose level over the 30 day period.
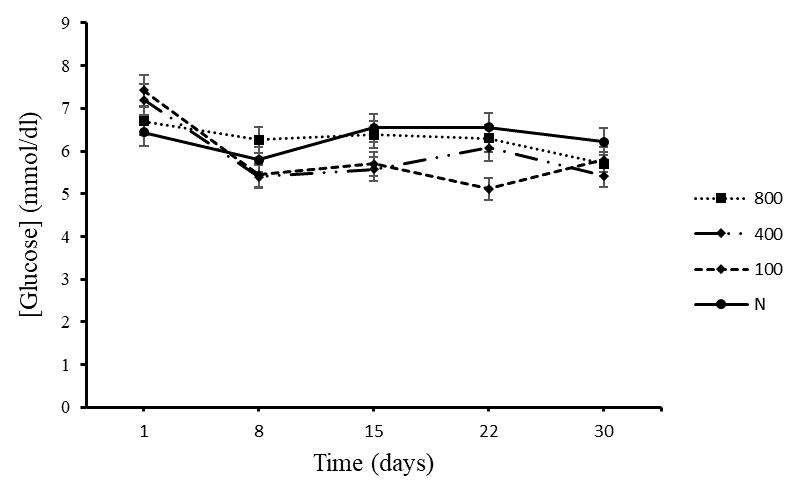
Figure 4.3. Blood glucose levels of the control groups that were given crude aqueous extract of Vitex payos and distilled water.
Levels of Malondialdehyde (MDA)
All diabetic rats under different treatments and the normal rats were tested for concentrations of MDA after 30 days. The diabetic rats treated with distilled water showed the greatest amount of MDA present as shown in Figure 4.4, and the normal control group on 400mg/kg treatment showed the least concentration.
Significance level was tested at 5%. There was no significant difference in MDA levels among the diabetic treated groups (ANOVA: single factor, P = 0.212). However, the diabetic group treated with distilled water had a higher level of MDA than the normal group on 400mg/kg aqueous extract (Two-tailed t-test, P = 0.0037) and the normal group on 800mg/kg (Two-tailed t-test, P = 0.0035).
The results are given as mean ± SD for the rats in each specific group.

Figure 4.4. Levels of MDA for diabetic rats on treatment with different concentrations of V. payos aqueous fruit extract, glibenclamide and distilled water. The normal control group F was treated with distilled water and the rest of the normal control groups were treated with different concentrations of V. payos aqueous fruit extract.
Level of Catalase Activity
The level of catalase activity was highest in the distilled water group as shown in figure 5. There was no significant difference in catalase activity among the control groups and those treated with aqueous extract of the fruit of V. payos and glibenclamide (ANOVA: single factor, p>0.05). The group treated with distilled water showed a significantly greater catalase activity than the control groups on V. payos aqueous fruit extract (t-Test: Two-tailed, P = 0.0044). There was also a significantly greater catalase activity for the group treated with distilled water as compared to the diabetic groups treated with both glibenclamide and aqueous extract of the fruit of V. payos (t-Test: Two-tailed, P = 0.008).
The results are given as mean ± SD for the rats in each specific group.
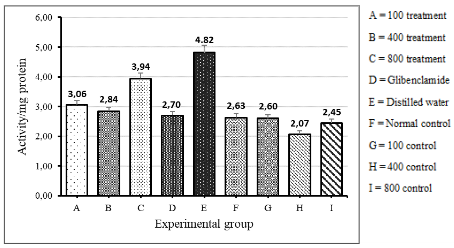
Figure 4.5. Catalase activity in diabetic groups treated with aqueous extract of fruit of V. payos, glibenclamide and distilled water (groups A to E). The catalase activity of normal rats on distilled water and aqueous extract of fruit of V. payos are also shown (groups F to I).
Levels of SOD activity
The level of SOD activity was also tested after 30 days of treatment. There was a significant difference in SOD activity among the diabetic and normal rat groups (ANOVA: single factor, P=0.00018).
The diabetic rats treated with distilled water showed the least amount of activity of SOD. There was no statistically significant difference of SOD activity between the diabetic rat group treated with 800mg/kg V. payos fruit extract and the normal control group treated with 800mg/kg V. payos fruit extract (t-Test: Two-tailed, P>0.05).
However, there was a significantly greater SOD activity in the diabetic rat group treated with 800mg/kg V. payos aqueous fruit extract compared to the diabetic rat group treated with distilled water (t-Test: Two-tailed, P = 0.036).
The SOD activity gradually increased in the diabetic treatment group as the concentration of V. payos aqueous fruit extract was increased from 100mg/kg to 400mg/kg to 800mg/kg as shown in Figure 6. The same trend was observed in the normal control groups treated with V. payos extract.
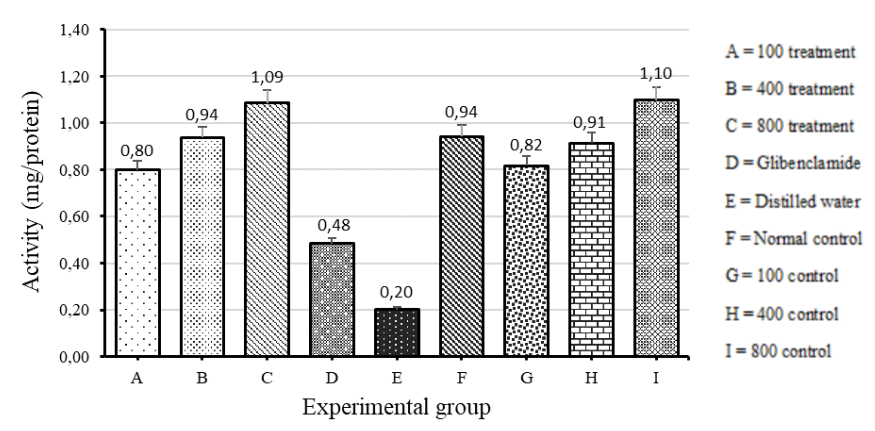
Figure 4.6. The level of SOD activity measured from the livers of the rats in the different experimental groups. The results are shown as mean ± SD for each group.
AST and ALT levels
A liver function test was performed on all the experimental groups after 30 days of treatment. The diabetic group treated with glibenclamide showed the least concentrations of both AST and ALT. The diabetic groups treated with V. payos aqueous fruit extract showed relatively higher concentrations of AST and ALT compared to all other groups.
On comparison of the means (±SD) for all groups, there was no significant difference in the concentrations of AST and ALT (ANOVA: single factor, P = 0.085). However, there was a generally lower concentration of the enzymes in the normal groups treated with V. payos aqueous fruit extract and glibenclamide (figure 8) as compared to the diabetic groups on V. payos aqueous fruit extract.
There was statistically no significant difference in the concentration of AST and ALT between the diabetic group treated with distilled water and the normal group treated with distilled water (t-Test: Two-tailed, P = 0.63). Only one result for glibenclamide was used, as the other 2 results had insufficient amount of sample for analysis.
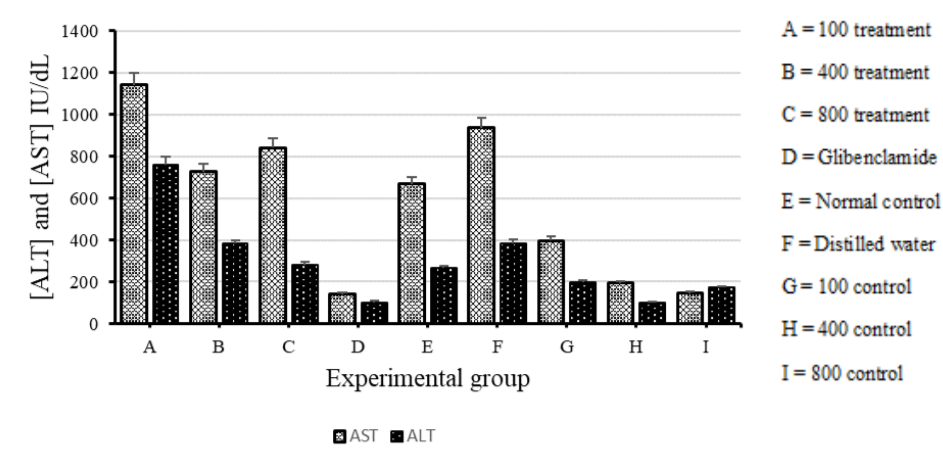
Figure 4.8. A comparison of the mean (±SD) AST and ALT levels of all experimental groups as determined by liver function tests performed after 30 days of treatment.
Discussion
The findings from this study suggest that the aqueous fruit extract of Vitex payos has a positive effect on lipid peroxidation and oxidative stress in alloxan-induced diabetic rats. This was evidenced from the various experiments that were conducted during the course of the study where several parameters were used to quantify and qualify the results.
In general, Sprague Dawley rats used for assessing antidiabetic effects of various compounds in various published papers weighed in the range 170 ± 30g. In this experiment the male Sprague Dawley rats on the high fat and sugar diet initially weighed 209.1 ± 51.12g (figure 1). This may have counted for the initial slow rate of growth as they were now older rats with less metabolic activity than the younger smaller rats. In comparison, the rats on standard rat feed showed a faster rate of growth initially and this could be attributed to their age as they were much younger than the other group. Both types of feed showed a gradual increase in weight over the 30 days, but the main difference was observed during the first 10 days of observation.
In order to cause diabetes in experimental rats, alloxan monohydrate was administered, and its mechanism includes the pancreatic beta cells partially degrading, which limits the quality and quantity of insulin generated (Dunn and McLetchie, 1943). This was evident in the diabetic rats treated with distilled water which showed a gradual increase in blood glucose level over the 30 days of treatment as there was little to no insulin to reverse the hyperglycemic state. On the other hand, glibenclamide (a sulfonylurea antidiabetic drug use for T2DM) helps reduce the amount of sugar in the blood by increasing the amount of insulin produced in the pancreas. In our experiment the group treated with glibenclamide showed the greatest rate of reduction of blood glucose over the 30 days of treatment, followed by the group on 800mg/kg aqueous V. payos fruit extract. This suggests that this concentration of extract boosts insulin production. The 100mg/kg and 400mg/kg did not show a marked improvement in reducing blood glucose suggesting that these concentrations are not as effective in boosting insulin production and or increasing insulin sensitivity. That means that the effect of the extract is dose dependent.
The data obtained from the control groups (figure 3) also showed that aqueous V. payos fruit extract has a hypoglycemic effect as over 30 days the normal rats on distilled water showed a relatively higher blood glucose level than the control groups on the different extract concentrations. This could be attributed to the effect of the extract on boosting production of insulin or increasing the sensitivity of the insulin receptors.
Previous studies have shown that hyperglycemia is the major cause for increased free radical levels which is followed by production of ROS. This results in elevated lipid peroxidation and a modified antioxidant defense mechanism, which further compromises the biological system's ability to metabolize glucose (Balasubashini et al., 2004). One of the products of lipid peroxidation is MDA which is considered its main biological marker. Thiobarbituric acid reactive substances (TBARS) are currently regarded as a reliable indicator of oxidative stress and lipid peroxidation (Tsai and Huang, 2015). In the study done, the diabetic group treated with distilled water showed a relatively higher level of MDA than all other groups. This could be because of a higher state of oxidative stress where the number of ROS exceeds the antioxidant condition caused by diabetes. When ROS increase it results in more lipid peroxidation and hence more MDA is produced.
The diabetic groups treated with the aqueous V. payos fruit extract showed no significant difference in levels of MDA concentration as compared to the group treated with distilled water. It suggests that the extract did not have a marked effect on reducing lipid peroxidation and thus more studies have to be done to ascertain this trend. As expected, the normal controls had a relatively lower concentration of MDA compared to both the treated groups on V. payos aqueous fruit extract and that treated with distilled water.
The enzymes SOD, CAT and reduced glutathione peroxidase (GSH-Px) are regarded as the first line of antioxidant defense system against the ROS generated in vivo during oxidative stress. They work very closely in the several metabolic pathways of free radicals (Cheng and Kong, 2011). Hayden and Tyagi (2002) documented that in DM, there is an increased generation of ROS, impaired formation of endogenous antioxidants such as SOD, reduced glutathione, reduced activity of peroxidase and catalase. This is because antioxidant enzymes are damaged by protein glycation. Bayraktutan (2002) also said that peroxidase enzyme scavenger activity is reduced in endothelial cells exposed to high concentration of glucose. This would suggest an overall reduction in the activity of all first line antioxidant enzymes. However, in this study it was discovered that CAT activity was actually increased in the diabetic group treated with distilled water (figure 5). The rest of the groups (extract treated groups and control groups) did not show any statistically significant difference in CAT activity. Bayraktutan (2002) discovered that biosynthesis and activity of CAT is increased in hyperglycemia to neutralize enhanced oxidant stress. Selvam et al. (1998) also said that increased CAT activity is due to the body trying to overcome the damaging effect of erythrocyte membrane oxidative attack.
The unexpected result of CAT activity in the diabetic group treated with distilled water shows how the interesting discovery can be explained through the study by Selvam et al. (1998).
SOD is part of the first line of defense against free radicals (Hamden et al., 2009) thus its activity would be affected first during the condition of oxidative stress. Hyperglycemia is accompanied by loss of Cu2+ which is essential cofactor in SOD activity. Thus, it was expected as observed in figure 6 that SOD activity would be least in the diabetic group treated with distilled water and highest in the control groups.
An interesting trend was the gradual increase in SOD activity observed in the diabetic groups treated with aqueous V. payos fruit extract. The activity increased gradually from the 100mg/kg, 400mg/kg and finally the 800mg/kg fruit extract. This suggests that the aqueous V. payos fruit extract has an effect of increasing the production of antioxidant enzymes or reversing the protein glycation caused by hyperglycemia. Similarly in the normal control groups on fruit extract, a trend was observed in the increase in SOD activity which would suggest that the fruit extract boosts the production of SOD.
The level of peroxide enzymes after 30 days was least in the diabetic group treated with distilled water. It was highest in the diabetic group treated with 800mg/kg of aqueous V. payos fruit extract and this was almost similar to the normal group on 100mg/kg fruit extract. The indication from this study is that hyperglycemia reduces the concentration of available peroxide enzymes involved in antioxidant activity. This can be improved by treating with 800mg/kg aqueous V. payos fruit extract (figure 7). Robertson (2004) showed that antioxidant enzymes halt the metabolic deterioration caused by diabetes by improving β-cells function in animal models and suggested that diabetes can be managed by enhancing antioxidant defense mechanisms in the pancreatic islets as a valuable pharmacological approach. Hence, the V. payos aqueous fruit extract may function by enhancing these mechanisms so that the animals can cope better with oxidative stress. The greater the production of antioxidant enzymes, the greater the levels of free radical scavenging.
When liver function tests are done, the biomarkers normally considered to ascertain the level of liver damage is the measurement of the concentration of the enzymes ALT and AST. Although being established quantitative markers of liver damage, ALT is the predominant enzyme found in the liver, whilst AST is found in the liver and muscle including the brain. When the liver becomes damaged, it leaks these enzymes into the blood by a process called transaminitis which can be detected in the blood plasma. The results from this experiment showed elevated levels of both AST and ALT in the blood plasma samples collected. However, it was expected that the normal control would have a much lower concentration of the two enzymes as compared to the diabetic group treated with distilled water. This was not observed as some of the samples used for analysis had lysed red blood cells. Ellinger et al. (2011) reported that both ALT and AST are found in human erythrocytes where they are vital for the production of glutamate for glutathione synthesis. This means that when a plasma sample contains lysed red blood cells, this increases the concentration of AST and ALT hence the assessment of liver damage due to diabetes becomes biased.
Interestingly was the trend observed of a decrease in ALT from the 100mg/kg dose to the 800mg/kg, which suggests that the higher dose repaired the liver damage more.
It is thus critical in this experiment that strictly the yellow coloured plasma samples be used to quantify the amount of ALT and AST so as to come up with a more accurate analysis of the extent of liver damage due to T2DM.
Conclusion
The findings in this experiment demonstrated the importance of plant extracts as pharmacological supplements to diseases such as Diabetes Mellitus. Vitex payos aqueous fruit extract lowered the blood glucose level in hyperglycemic alloxan induced diabetic rats and did not show any significant change in the normal level of glucose in healthy rats. It also did not show any toxic effects in vivo. This finding provides strong scientific evidence that Vitex payos fruit extract has a strong antidiabetic potential which justifies its use as a food supplement or an adjunct in the management of diabetes and other associated complications.
Although more work still needs to be done in studying the effect of the fruit extract on insulin sensitivity or production of insulin by repairing β-cells of the pancreas, the ability of the fruit extract to boost production of antioxidant enzymes is valuable in suggesting its use in management of diabetes and its secondary complications.
The study also revealed the dose dependent effect of aqueous V. payos fruit extract in antidiabetic activity, blood glucose level and antioxidant activities. The findings also suggested that the aqueous fruit extract at dose 800mg/kg had a more significant blood glucose lowering effect and greater antioxidant enzyme activity. This gives it the potential to be used in the development of herbal formulations for control of diabetes mellitus and its clinical complications.
The availability of less expensive protocol methods of assaying the quantity of the antioxidant enzymes studied may allow more fruit extracts to be studied for their efficacy in managing diabetes mellitus.
In future work, further studies could be conducted on lipid lowering properties and effect on increasing levels of insulin production.
BIBLIOGRAPHY
[1] Agbafor, K.N., Nwachukwu, N., 2011. Phytochemical Analysis and Antioxidant Property of Leaf Extracts of Vitex doniana and Mucuna pruriens. Biochemical Research International, vol. 2011.
[2] Alarcon-Aguilara, F.J., Ramon-Ramos, R., Perez-Gutierrez, S., Aguilar-Contreras, A., Contreras-Weber, C.C., and Flores-Saenz, J.L., 1998. Study of the anti-hyperglycemic effect of plants used as anti-diabetics. Journal of Ethnopharmacology, 61, pp 101-110.
[3] Atanasov, A.G., et al., 2015. Discovery and resupply of pharmacologically active plant- derived natural products: A review,Biotechnology Adv.
[4] Bailey, C.J., and Day, C., 1989. Traditional plant medicines as treatments for diabetes. Diabetes Care, pp 553-564.
[5] Balasubashini, M.S., Rukkumani, R., Viswanathan, P., Venugopal, P.M., 2004. Ferulic acid alleviates lipid peroxidation in diabetic rats. Physiotherapy research: PTR, pp 310-314.
[6] Balasundram, N., Sundram, K., Samman, S., 2006. Phenolic compounds in plants and agri- industrial by-products. Antioxidant activity, occurrence, and potential uses, Food Chem, pp 191–203.
[7] Bayraktutan, U., 2002. Free radicals, diabetes, and endothelial dysfunction. Diabetes Obes Metab, 224–238.
[8] Brownlee, M., 2001. Biochemistry and molecular cell biology of diabetic complications. Nature 414, pp 813–820.
[9] Cheng, D., and Kong, H., 2011. The effect of Lycium barbarum polysaccharide on alcohol induced oxidative stress in rats. Molecules, 16(3), pp 2542-2550.
[10] Costacou, T., and Mayer-Davis, E.J., 2003. Nutrition and prevention of type 2 diabetes Ann. Rev. Nutrition, pp 147–170.
[11] Defronzo, R.A., 1999. Pharmacologic therapy for type 2 diabetes mellitus. Ann Intern Med.
[12] Dianzani, M., and Barrera, G., 2008. Pathology and physiology of lipid peroxidation and its carbonyl products. Torino, Italy, Ref 115, pp 19-38.
[13] Dunn, J.S., and McLetchie, N.G.B., 1943. Experimental Diabetes In The Rat. The Lancet, Vol 242, pp 384-387.
[14] Ellinger, J.J., Lewis, I.A., Markley, J.L., 2011. Role of aminotransferases in glutamate metabolism of human erythrocytes. Journal of Biomolecular NMR, pp 221-229.
[15] Engelen, W., Manuel-y-Keenoy, B., Vertommen, J., De Leeuw, I., Van Gaal, L., 2005. Effects of micronized fenofibrate and vitamin E on in vitro oxidation of lipoproteins in patients with type I diabetes. Diabetes metabolism, pp 197-204.
[16] Evans, J.L., Goldfine, T.D., Maddux, B.A., Grodsky, G.D., 2002. Oxidative stress and stress-activated signaling pathways. A unifyng hypothesis of type 2 diabetes, Endocr Rev pp 599–622.
[17] Foote, C.S., 1996. Autoxidation. Active Oxygen in Chemistry, Dordrecht: Springer, Netherlands, pp 24–65.
[18] Fritz, K.S., and Petersen, D.R., 2013. An overview of the chemistry and biology of reactive aldehydes. Free Radic. Biol. Med, pp 85–91.
[19] Gohdes, D., 1995. Diabetes in North American Indians and Alaska Natives. In Diabetes in America, 8th edition, US Department of Health and Human Services. DHHS publication.
[20] Guo, W., Adachi, T., Matsui, R., Xu, S., Jiang, B., Zou, M.H., Kirber, M., Lieberthal, W., Cohen, R.A., 2003. Quantitative assessment of tyrosine nitration of manganese superoxide dismutase in angiotensin II-infused rat kidney. Am. J. Physiol. Heart Circ. Physiol.
[21] Hamden, K., Carreau, S., Jamoussi, K., Miladi, S., Lajmi, S., Aloulou, D., 2009. 1Alpha, 25 dihydroxyvitamin D3: therapeutic and preventive effects against oxidative stress, hepatic, pancreatic and renal injury in alloxan-induced diabetes in rats. J Nutr Sci Vitaminol, pp 215–222.
[22] Hayden, M.R., and Tyagi, S.C., 2002. Intimal Redox Stress: Accelerated Atherosclerosis in Metabolic Syndrome and Type 2 Diabetes Mellitus. Journal of Atheroscleropathy, Cardiovasculogy and Diabetology, pp 3-8.
[23] Hayden, M.R., and Tyagi, S.C., 2002. Islet redox stress: the manifold toxicities of insulin resistance. Metabolic syndrome and amylin derived islet amyloid in type 2 diabetes mellitus, pp 86–108.
[24] Helenoa, S.A., Martins, A., Queiroz, M.J.R.P., Ferreira, I.C.F.R., 2015. Bioactivity of phenolic acids. Metabolites versus parent compounds: A review, Food Chem, pp 501– 513.
[25] Hung, H.C., Joshipura, K.J., Jiang, R., 2004. Fruit and vegetable intake and risk of major chronic disease. J. Natl. Cancer Inst, pp 1577–1584.
[26] Iwai, K., 2008. Antidiabetic and antioxidant effects of polyphenols in brown alga Ecklonia stolonifera in genetically diabetic KK-A(y) mice. Plant Foods Hum. Nutrition, pp 163– 169.
[27] Jamison, D.T., 2006. Disease and Mortality in Sub-Saharan Africa. World Bank Publications.
[28] Kelly, D.E., 1995. Effects of weight loss on glucose homeostasis in NIDDM. Diabetes Rev.
[29] Kim, J., Sohn, E., and Kim,C.S., 2012. In vivo anti-diabetic effect of aster korainsesis extract. Plant Med, 78(11): PD99.
[30] Kim, S.H., Hyun, S.H., Choung, S.Y., 2006. Anti-diabetic effect of cinnamon extract on blood glucose in db/db mice. Journal of Ethnopharmacology, Vol 104, pp 119-123.
[31] Li, N., Frigerio, F., Maechler, P., 2008. The sensitivity of pancreatic β-cells to mitochondrial injuries triggered by lipotoxicity and oxidative stress. Biochem Soc Trans.
[32] Lin, D., Mengshi, X., Jingjing, Z., Zhuohao, L., Baoshan, X., Xindan, L., Maozhu K., Liangyu, L., Qing, Z., Yaowen, L., Hong, C., Wen, Q., Hejun, W., and Saiyan. C., 2016. An Overview of Plant Phenolic Compounds and Their Importance in Human Nutrition and Management of Type 2 Diabetes. Molecules 21, no. 10: 1374.
[33] Matamoros, M.A., Dalton, D.A., Ramos, J., Clemente, M.R., Rubio, M.C. Becana, M., 2003. Biochemistry and molecular biology of antioxidants in the rhizobia–legume symbiosis. Plant Physiology, pp 133:499–509.
[34] McCord, J.M., 2000. The evolution of free radicals and oxidative stress. Am. J. Med, pp 652–659.
[35] McDougall, G.J., Shpiro, F., Doboson, P., Smith, P., Blake, A., Stewart, D., 2005. Different polyphenolic compounds of soft fruits inhibit α-amylase and α-glucosidase. J. Agric. Food Chem.
[36] Meigs, J.B., 2009. Multiple biomarker prediction of type 2 diabetes. Diabetes Care. New insights on aging and aging-related diseases from eukaryotic and prokaryotic model organisms. Oxid. Med. Cell. Longev.
[37] Olokoba, A.B., Obateru, O.A., Olokoba, L.B., 2012. Type 2 diabetes mellitus: a review of current trends. Oman Med J. 27(4), pp269-73.
[38] Parveen, A., Parveen, B., Parveen, R., Ahmad, S., 2015. Challenges and guidelines for clinical trial of herbal drugs. J Pharm Bioallied Science. Oct-Dec, pp 329-33.
[39] Patwardhan, B., 2005. Ethnopharmacology and drug discovery. Journal of Ethnopharmacology, Vol 100, Issues 1-2, pp 50-52.
[40] Pfeiffer, A.F., and Klein, H.H., 2014. The treatment of type 2 diabetes. Dtsch Arztebl Int. 111(5), pp 69-81;
[41] Pinto, M.S., Lajolo, F.M., Genovese, M.I., 2007. Bioactive compounds and antioxidant capacity of strawberry jams. Plant Foods Hum. Nutr, 62, pp 127–131.
[42] Poitont, V. and Robertson R.P., 2008. Glucolipotoxicity: fuel excess and β-cell dysfunction. Endocr Rev.
[43] Pu, F., Ren, X.L., Zhang, X.P., 2013. Phenolic compounds and antioxidant activity in fruits of six Diospyros kaki genotypes. Eur. Food Res. Technology, pp 923–932.
[44] Radi, R., Beckman, J.S., Bush, K.M., Freeman, B.A., 2013. Peroxynitrite, a stealthy biological oxidant. J. Biol. Chem.
[45] Randhir, R., Lin, Y.T., Shetty, K., 2004. Stimulation of phenolics, antioxidant and antimicrobial activities in dark germinated mung bean sprouts in response to peptide and phytochemical elicitors. Process Biochem, 39, pp 637–646.
[46] Rehman, S., Harris, P.J.C., Ashraf, M., 2005. Stress environments and their impact on crop production. In Abiotic Stresses: Plant Resistance through Breeding and Molecular Approaches, Haworth Press, New York, NY, USA, pp 3-18.
[47] Robertson, R.P., 2004. Chronic Oxidative Stress as a Central Mechanism for Glucose Toxicity in Pancreatic Islet Beta Cells in Diabetes. Journal of Biomolecular Chemistry.
[48] Santos, A. L., Sinha, S., and Lindner, A. B., 2018. The good, the bad, and the ugly of ROS:
[49] Schlichtmann, J., and Graber, M.A., 1997. Hematologic, Electrolyte, and Metabolic Disorders. In: Graber MA, Toth PP, Herting RL, eds, The Family Practice Handbook. 3rd edn, St. Louis, Missouri: Mosby-Yearbook Inc, pp 192- 251.
[49] Seeram, N.P., 2008. Berry Fruits: Compositional Elements, Biochemical Activities, and the Impact of Their Intake on Human Health, Performance, and Disease. Journal of Agricultural and Food Chemistry, 56, pp 627- 629.
[50] Seid, M.A, Akalu, Y., Gela, Y.Y., 2021. Microvascular complications and its predictors among type 2 diabetes mellitus patients at Dessie town hospitals, Ethiopia. Diabetol Metab Syndrome.
[51] Selvam, R., and Anuradha, C.V., 1998. Lipid peroxidation and antiperoxidative enzyme changes in erythrocytes in diabetes mellitus. Indian J Biochem Biophys, pp 268–72.
[52] Sinha, A., Formica, C., Tsalamandris, C., 1996. Effect of insulin on body composition in patients with insulin-dependent and non-insulin dependent diabetes. Diabetes Med, pp 40-46.
[53] Song, Y.Q., Manson, J.E., Buring, J.E., Sesso, H.D., Liu, S., 2005. Associations of dietary flavonoids with risk of type 2 diabetes, and markers of insulin resistance and systemic inflammation in women. A prospective study and crosssectional analysis, J. Am. Coll. Nutr, pp376–384.
[54] Sookoian, S. and Pirola, C.J., 2015. Liver enzymes, metabolomics and genome-wide assocation studies: from systems biology to the personalised medicine. World J Gastroentererol.
[55] Tabatabaei-Malazy, O., Larijani, B., Abdollahi, M., 2012. A systematic review of in vitro studies conducted on effect of herbal products on secretion of insulin from Langerhans islets. J Pharm Pharm Sci, pp 447-466.
[56] Taderera, T., Gomo, E., and Chagonda, L.S., 2014. The Antidiabetic Activity of an Aqueous Root Extract of Annona stenophylla Engl. and Diels in Non-diabetic Control and Alloxan-induced Diabetic rats. Journal of Biologically Active Plants from Nature, pp 315-322.
[57] Tilton, W.M., Seaman, C., Carriero, D., Piomelli, S.,1991. Regulation of glycolysis in the erythrocyte. Role of the lactate/pyruvate and NAD/NADH ratios. J Lab Clin Med, pp 146–52.
[58] Tsai, M.C., and Huang, T.L., 2015. Thiobarbituric acid reactive substances (TBARS) is a state biomarker of oxidative stress in bipolar patients in a manic phase. Journal of Affective Disorders, Volume 173, pp 22-26.
[59] Tsuda, T., 2008. Regulation of adipocyte function by anthocyanins. Possibility of preventing the metabolic syndrome. J. Agric. Food Chem, pp 642–646.
[60] Vanaclocha, B., Risco, E., Cañigueral, S., 2014. Interacciones entre preparados vegetales y fármaos de sínteis. Revisión de las monografías de la EMA y ESCOP, Rev Fitoter, pp 5- 36.
[61] Whiting, D.R., Guariguata, L, Weil, C., Shaw, J., 2011. IDF diabetes atlas: global estimates of the prevalence of diabetes for 2011 and 2030. Diabetes Res Clinical Practice.
[62] Wild, S., Roglic, G., Green, A., Sicree, R., King, H., 2004. Global prevalence of diabetes: Estimates for year 2000 and projections for 2030. Diabetes Care, pp 1047-53.
[63] Wolff, S.P., Jiang, Z.Y, and Hunt, J.V., 1991. Protein glycation and oxidative stress in diabetes mellitus and ageing. Free Radic Biol Med, pp 339–352.
[64] Yeh, G.Y., Eisenberg, D.M., Kaptchuk, T.J., Phillips, R.S., 2003. Systematic review of herbs and dietary supplements for glycemic control in diabetes. Diabetes Care, pp 1277– 1294.
[65] Zheng, Y., Li, X.K., Wang, Y., 2008. The role of Zinc, Copper, and Iron in the pathogenesis of diabetes and diabetic complications. Therapeutic effects by chelators: Hemoglobin.
Comments